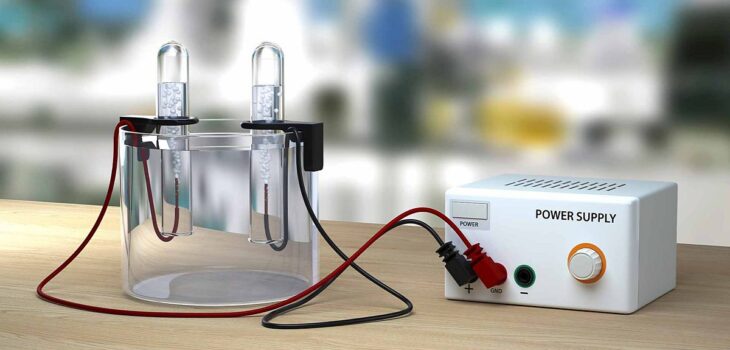
Transforming anion exchange membranes in water electrolysis for green hydrogen production
What Are the Economic Implications of Advancements in Anion Exchange Membranes for Green Hydrogen Technologies?
Water electrolysis, a process that involves the splitting of water (H₂O) into hydrogen (H₂) and oxygen (O₂) by applying an electrical current, has gained substantial attention as a promising method for green hydrogen production. Green hydrogen, produced through renewable energy sources such as wind, solar, or hydropower, is considered a key component in the global transition towards sustainable energy. One of the crucial advancements in water electrolysis technology is the development of efficient and durable ion-exchange membranes, particularly anion exchange membranes (AEMs), which hold the potential to significantly enhance the performance and economics of water electrolysis for green hydrogen.
Anion exchange membranes are a type of polymer electrolyte membrane (PEM) that conduct hydroxide ions (OH⁻) rather than protons (H⁺), distinguishing them from the more commonly known proton exchange membranes (PEMs) used in hydrogen fuel cells. The role of AEMs in water electrolysis is to separate the anode and cathode compartments while allowing the flow of hydroxide ions from the cathode to the anode. This flow of hydroxide ions is essential for maintaining electrical neutrality during the electrolysis process. The development and optimization of AEMs are critical in improving the efficiency, stability, and cost-effectiveness of water electrolysis systems.
Challenges in Anion Exchange Membrane Development
According to a Water Electrolysis Market report, the industry is expected to grow significantly in the coming years. Although AEMs offer several advantages over traditional PEMs in water electrolysis, such as lower material costs and the ability to operate at higher current densities, their development has faced several challenges. One of the primary challenges is the relatively low conductivity of hydroxide ions compared to protons, which limits the performance of AEMs in high-efficiency electrolysis applications. Additionally, the stability of AEMs is another major concern. In the harsh alkaline environment created during electrolysis, many AEMs are prone to degradation, which can significantly reduce their operational lifespan and increase the cost of hydrogen production.
To address these issues, researchers have focused on improving the ionic conductivity and chemical stability of AEMs. Several strategies have been proposed, including the development of new membrane materials, modifications to the polymer backbone, and the use of crosslinking agents to enhance membrane integrity. The incorporation of inorganic fillers, such as zirconium or titanium oxides, into the membrane structure has also been explored to improve ion conductivity and resistance to degradation.
Material Innovations for Improved AEM Performance
The key to advancing AEM technology lies in the development of novel materials that combine high ionic conductivity with excellent chemical stability. Traditional AEMs are often made from quaternary ammonium-based polymers, which, while effective in facilitating ion conduction, suffer from poor stability under high pH conditions. Recent research has focused on designing new polymer structures with more robust functional groups, such as imidazolium, phosphonium, and sulfonium, which offer enhanced stability in alkaline environments.
Another approach is the development of block copolymers, where different polymer blocks are designed to optimize ion conductivity and mechanical strength. These block copolymers are often functionalized with highly polar groups that promote the efficient transport of hydroxide ions. Furthermore, researchers have explored the use of perfluorinated sulfonic acid groups in combination with anion-exchange functionalities to create membranes with both high ionic conductivity and good chemical stability. These hybrid materials have shown promising results, balancing the need for high-performance membranes with durability in the aggressive electrolysis environment.
Enhancing the Durability of AEMs
Durability is a critical factor for the successful commercialization of AEM-based electrolysis systems. To extend the lifespan of AEMs, efforts have been made to improve their resistance to oxidative and hydrolytic degradation, which are common in alkaline electrolysis conditions. The oxidative stability of the membrane is particularly important, as hydroxide ions are highly reactive and can attack the polymer backbone, leading to degradation and performance loss. To mitigate this, researchers are developing AEMs that incorporate antioxidant materials, such as phenolic compounds or metal-based stabilizers, which help protect the membrane from oxidative attack.
Another strategy to enhance durability is the development of membrane-electrode assemblies (MEAs) with improved interfacial contact between the membrane and the electrodes. Effective electrode-membrane interactions are essential for ensuring that hydroxide ions can be efficiently transported through the membrane while minimizing the resistance to ion flow. Advances in MEA fabrication, such as the use of porous electrode materials and advanced membrane treatments, have resulted in significant improvements in the long-term stability and performance of AEM-based electrolyzers.
AEM Electrolyzers: Economic and Environmental Benefits
The potential benefits of AEM-based electrolyzers are not limited to performance improvements. One of the key advantages of AEMs over PEMs in water electrolysis is their cost-effectiveness. AEMs do not require the use of precious metals like platinum and iridium, which are essential for the catalysts in PEM electrolysis. This makes AEM-based systems more economically viable, particularly for large-scale hydrogen production. The reduced cost of the membrane materials, combined with the ability to use less expensive catalysts, could significantly lower the overall capital costs of electrolysis systems, making green hydrogen more competitive with hydrogen produced from fossil fuels.
Furthermore, the use of AEMs in water electrolysis aligns well with the goals of reducing the environmental impact of hydrogen production. Since AEMs enable the use of renewable energy sources for the electrolysis process, the resulting hydrogen is produced with minimal carbon emissions. This makes AEM-based electrolyzers a key technology in the transition to a low-carbon economy, particularly in sectors such as transportation, industrial manufacturing, and energy storage, where hydrogen can serve as a clean alternative to fossil fuels.
The development of anion exchange membranes for water electrolysis represents a significant step forward in the pursuit of green hydrogen production. While challenges related to ion conductivity, chemical stability, and durability remain, recent advancements in material science and membrane design are helping to address these issues. As AEM technology continues to evolve, it has the potential to revolutionize the economics and scalability of hydrogen production, making green hydrogen a more viable and sustainable energy solution for the future. By improving the performance and cost-effectiveness of water electrolysis systems, AEMs will play a crucial role in accelerating the global shift toward a hydrogen-based economy.